Currently, there is a growing need to improve the power performance of batteries, which would enable faster charging and improved performance of electronic devices. However, the internal kinetics of most batteries prevent the rapid transport of electrons and ions, which limits power density. We have developed hierarchical battery architectures and advanced manufacturing technologies to dramatically increase the power density of primary and secondary microbatteries by controlling ion and electron transport across nm – mm scales. We seek to further understand the limits of electron and ion transport, develop high power architectures for conventionally sized batteries, and dramatically improve the energy and power density of microbatteries.
High power microbatteries:
In this project we designed and fabricated hierarchical microbatteries with unprecedented power density. The three-dimensional bicontinuous interdigitated microbattery architecture improved power performance by simultaneously reducing ion and electron transport distances through the anode, cathode, and electrolyte. The microbattery power densities were up to 7.4 mW cm-2 mm-1, which equals or exceeds that of the best supercapacitors, is 100X greater than conventional batteries, and is 2000 times higher than that of other microbatteries. Electrochemical deposition techniques improved the microbattery energy density while maintaining high power density by allowing high volume fractions of electrochemically active material to be integrated into the high power architectures.
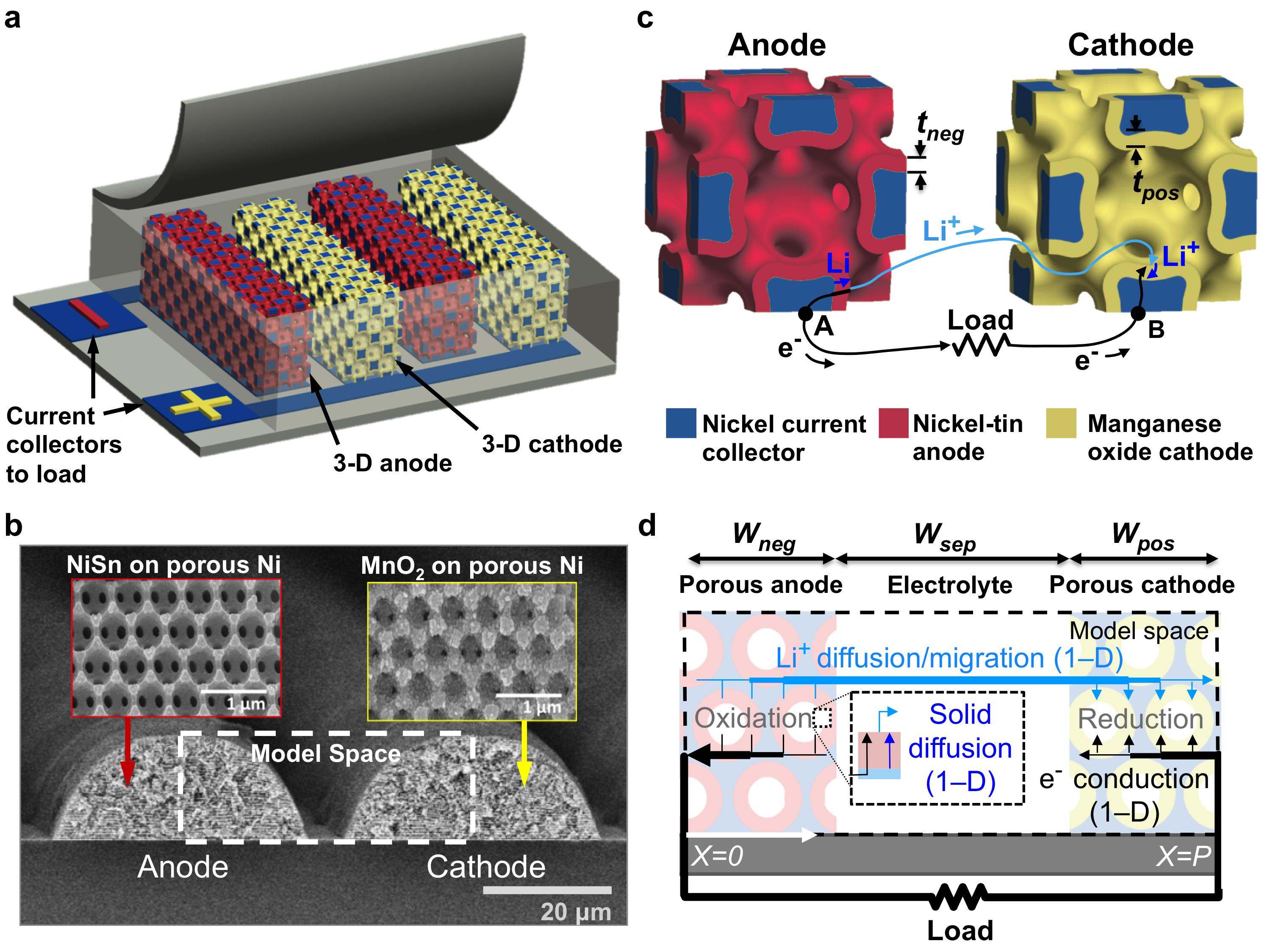
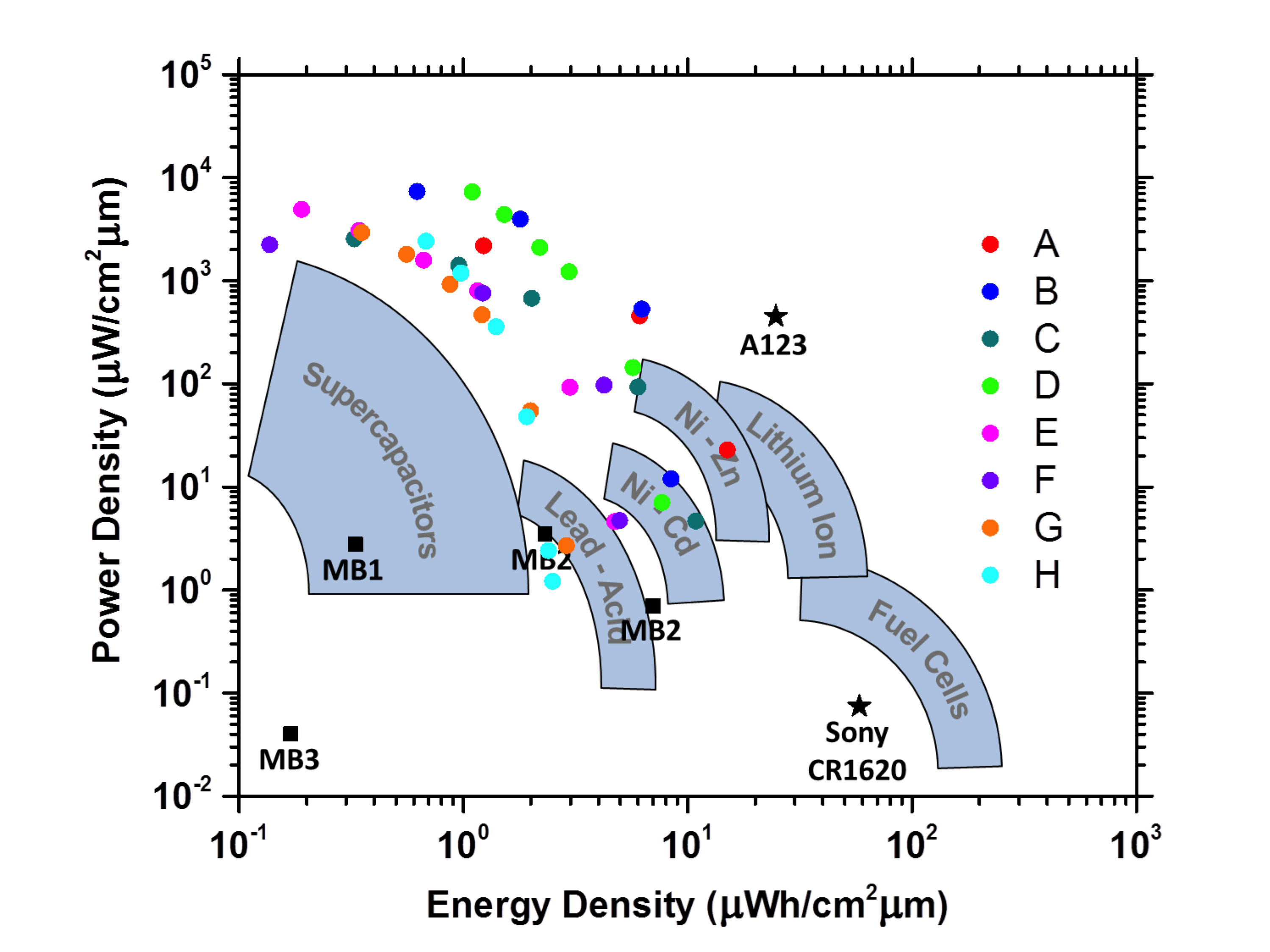
High power primary microbatteries:
In this project we developed technologies for integrating high volume fractions of high capacity materials into a primary microbattery. The primary microbatteries had similar energy densities to commercially available lithium/manganese oxide based primary batteries with a ~50 X higher peak power density.
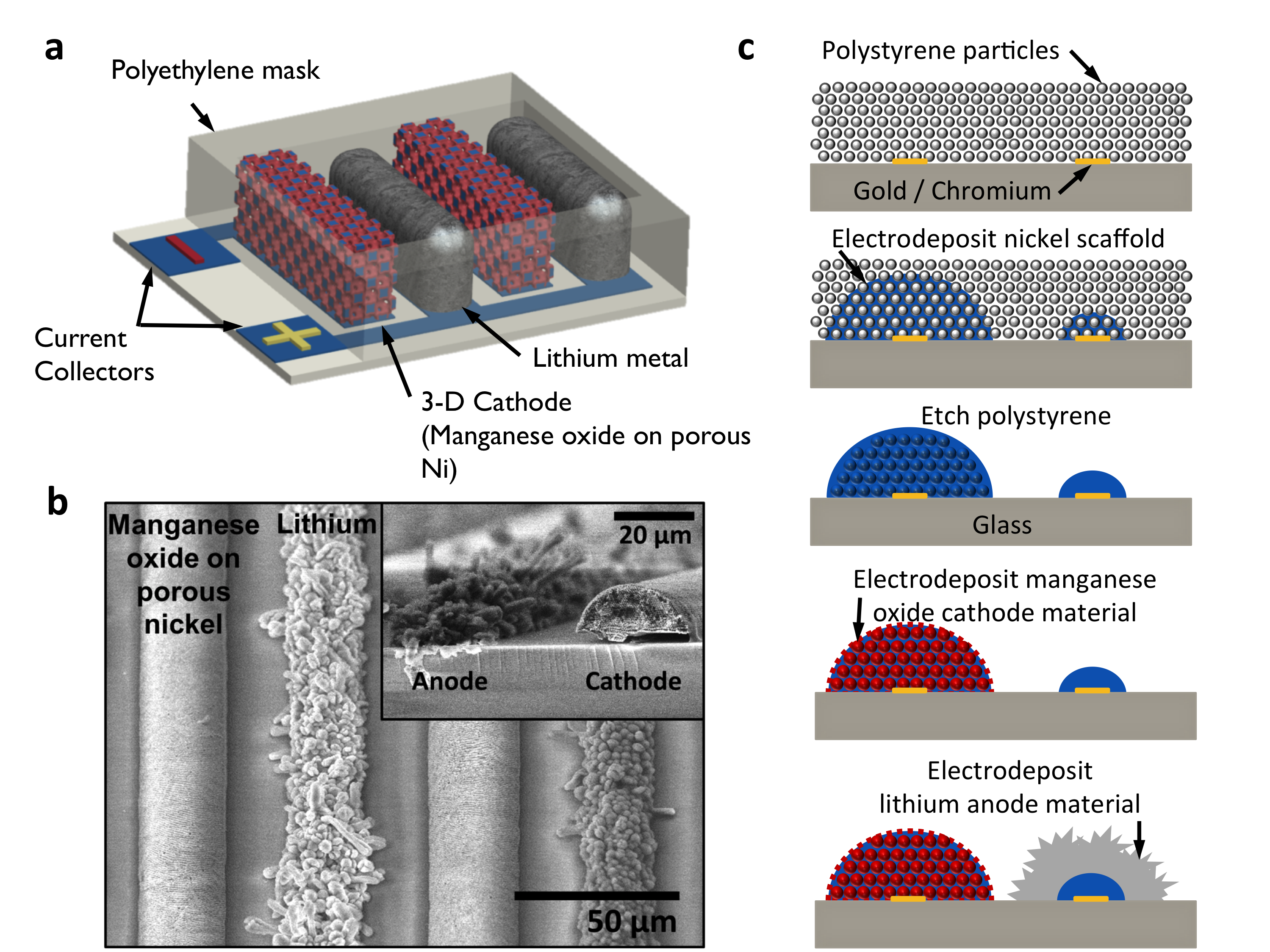
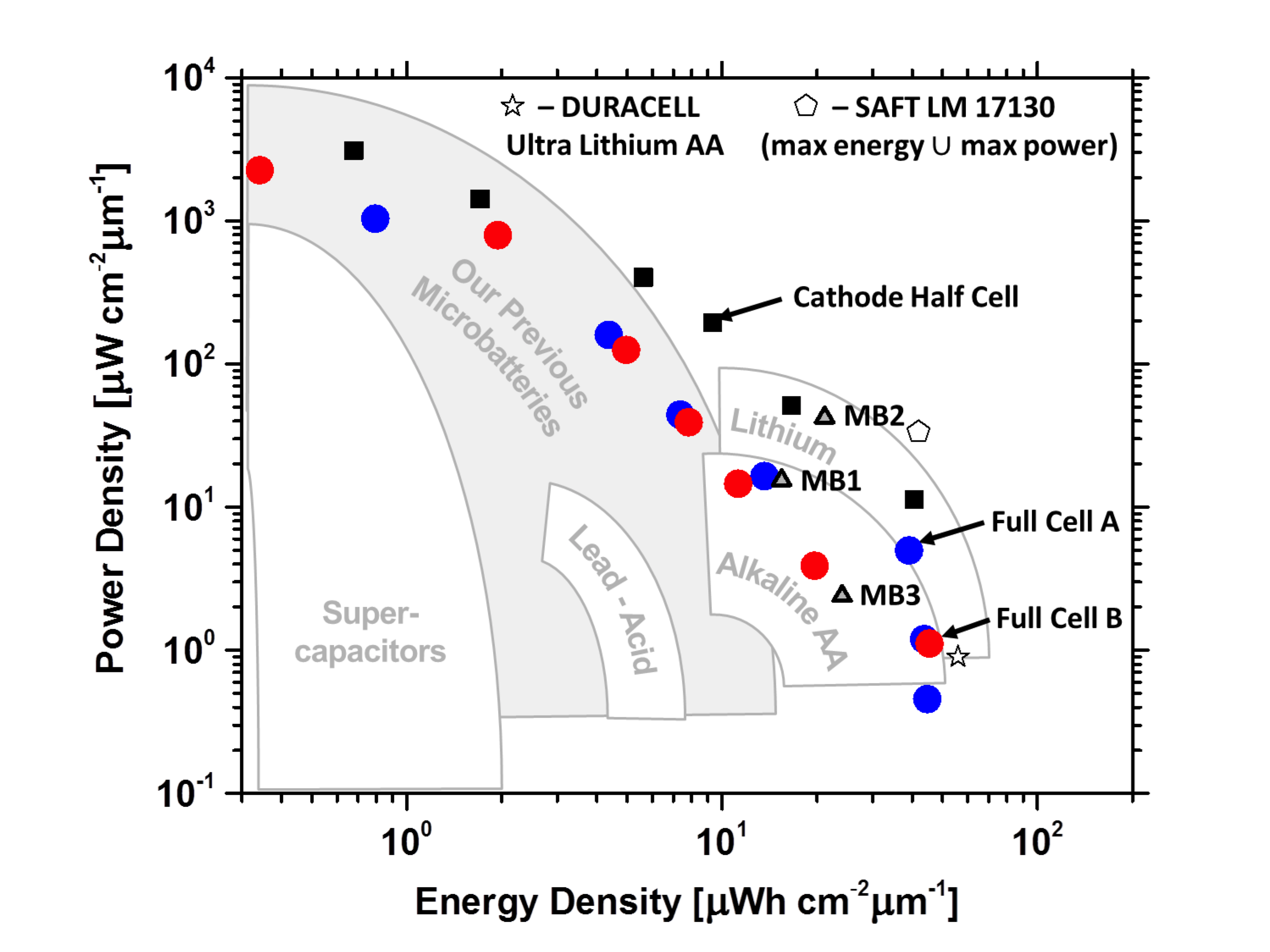
Holographic lithography for on-chip microbattery integration:
In this project we demonstrated a high-performance microbattery suitable for large-scale on-chip integration with both microelectromechanical and complementary metal-oxide–semiconductor (CMOS) devices. Enabled by a 3D holographic patterning technique, the battery possessed well-defined, periodically mesostructured porous electrodes. Such battery architectures offer both high energy and high power, and the 3D holographic patterning technique offers exceptional control of the electrode’s structural parameters, enabling customized energy and power for specific applications.
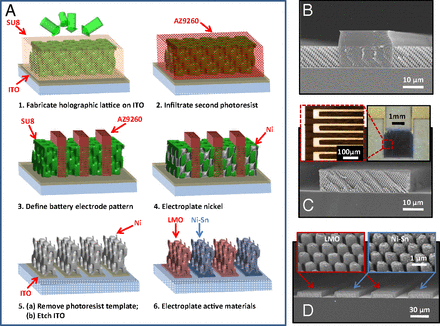
Publications:
[11] Xiujun Yue, Jessica Grzyb, Akaash Padmanabha, and James H. Pikul. “A Minimal Volume Hermetic Packaging Design for High-Energy-Density Micro-Energy Systems” Energies vol. 13, no. 10, pp. 2492, 2020.
[10] Michael Synodis, James Pikul, Sue Ann Bidstrup Allen, and Mark G. Allen. “Vertically integrated high voltage Zn-Air batteries enabled by stacked multilayer electrodeposition”, Journal of Power Sources, vol. 449 pp. 227566, 2020.
[9] Johnson, R. Kohlmeyer, M. N. Ates, C. Kiggins, A. Blake, J. B. Cook, J. H. Pikul, “Performance Modeling and Design of High Energy Density Microbatteries”, The 19th International Conference on Micro and Nanotechnology for Power Generation and Energy Conversion Applications (PowerMEMS), Krakow, 2019 [Best paper award]
[8] James H. Pikul, Jeffrey W. Long, “Architected materials for advanced electrochemical systems”, MRS Bulletin, vol. 44, no. 10, pp. 789-795, 2019.
[7] James H. Pikul, Hailong Ning, “Powering the internet of things”, Joule, vol. 2, pp. 1036–1038, June 20, 2018.
[6] James H. Pikul, Paul V. Braun, and William P. King. “Performance Modeling and Design of Ultra-High Power Microbatteries.” Journal of The Electrochemical Society, vol. 164.11, pp. E3122-E3131,2017.
[5] James H. Pikul, Jinyun Liu, Paul V. Braun, William P. King, “Integration of high capacity materials into interdigitated mesostructured electrodes for high energy and high power density primary microbatteries.” Journal of Power Sources, vol. 315, pp. 308-315, 2016.
[4] Hailong Ning, James H. Pikul, Runyu Zhang, Xuejiao Li, Sheng Xu, Junjie Wang, John A. Rogers, William Paul King, and Paul V. Braun, ” Holographic Patterning of High Performance on-chip 3D Lithium-ion Microbatteries”, Proceeding of the National Academy of Sciences, vol. 112, no. 21, pp. 6573-6578, 2015.
[3] Jinyun Liu, Huigang Zhang, Junjie Wang, Jiung Cho, James Pikul, Eric Scott Epstein, Xingjiu Huang, Jinhuai Liu, Paul V. Braun, “Hydrothermal Fabrication of Three-Dimensional Secondary Battery Anodes”, Advanced Materials, vol. 26, no. 41, pp. 7096-7101, 2014.
[2] James H. Pikul, Huigang Zhang, Jiung Cho, Paul V. Braun, and William P. King, “High power lithium ion micro batteries from interdigitated three-dimensional bicontinuous nanoporous electrodes”, Nature Communications, vol. 4, pp. 1732, 2013
[1] Paul V. Braun, Jiung Cho, James H. Pikul, William P. King, and Huigang Zhang, “High power rechargeable batteries”, Current Opinion in Solid State & Materials Science, vol. 16, pp. 186 – 198, 2012.