Hierarchical pore structures can dramatically change the mechanical and chemical properties of materials. We demonstrate methods for integrating hierarchical, nanoporous materials into microelectromechanical sensors to achieve unique mechanical properties in microcantilevers and improved sensing in ZnO glucose sensors.
3D porous microcantilevers:
In this project we demonstrate microcantilevers fabricated from regular 3D macroporous foams having a nickel inverse opal or alumina shell structure. The fabrication, based on an inverse-opal template process, offers 3D spatial control of the ordered foams with unique geometries and composite materials not achievable by subtractive fabrication. The mechanical properties of these devices depend upon the porosity, as expected, however the deformation modes are dramatically different, depending upon the micro-architecture. The Young’s moduli of the microcantilevers ranged from 2.0 to 44.3 GPa, which are comparable to materials ranging in stiffness from Nylon to high strength concrete or magnesium. At a 0.15 solids volume fraction, the Young’s moduli of the inverse opal and shell structures differ by a factor of 10. The ability to control the mechanical properties of a micromechanical device could unlock significant new opportunities in a variety of applications. The heirachical structure of the inverse opal and shell microcantilevers allows for the mechanical properties to be controlled, while the chemical, electrical, and other physical properties remain unchanged.
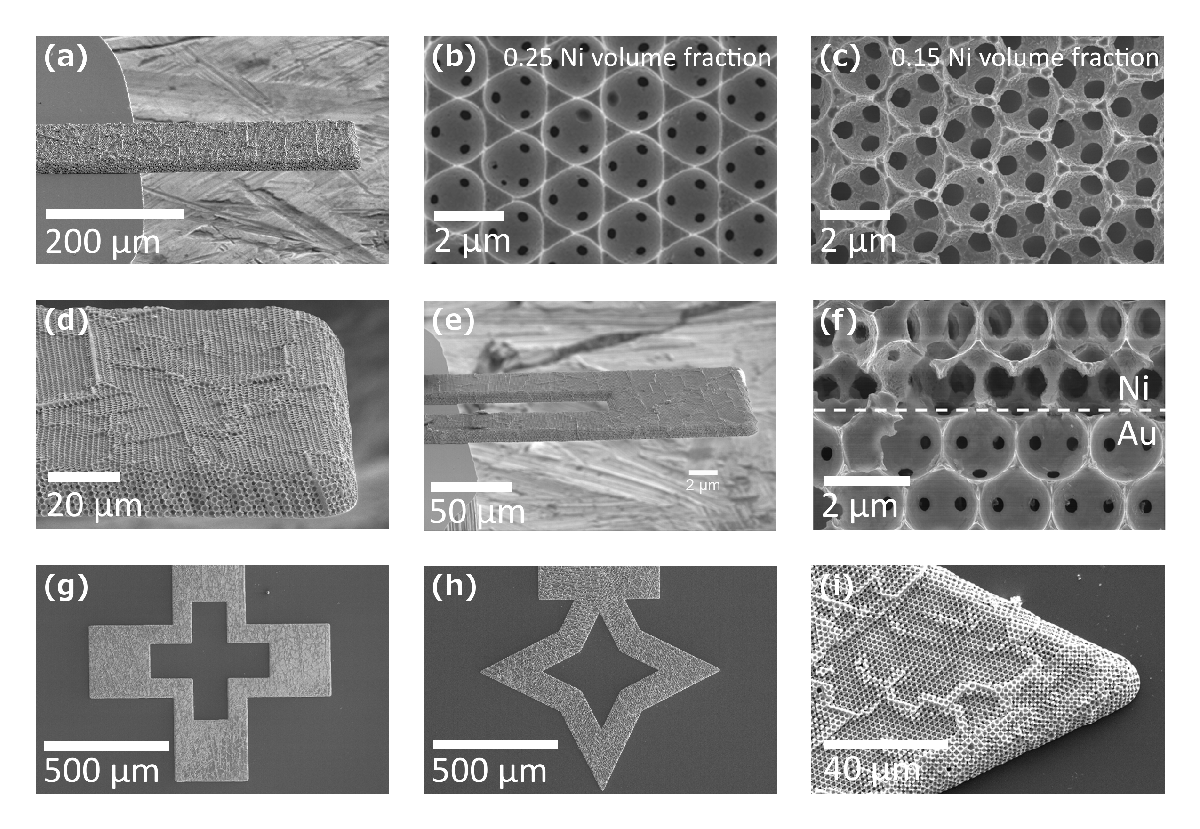

Micro ZnO glucose sensors:
In this work, ZnO inverse opal based enzymatic biosensors with linear detection ranges between 0.01 and 18 mM were developed. The ZnO inverse opal was chemically and mechanically robust, providing a suitable microenvironment for enzyme immobilization with long-term stability. The inverse opal geometry reduced the diffusivity of glucose, which extended the linear detection range by allowing diffusion-limited glucose oxidation on the ZnO, even at high bulk glucose concentrations.
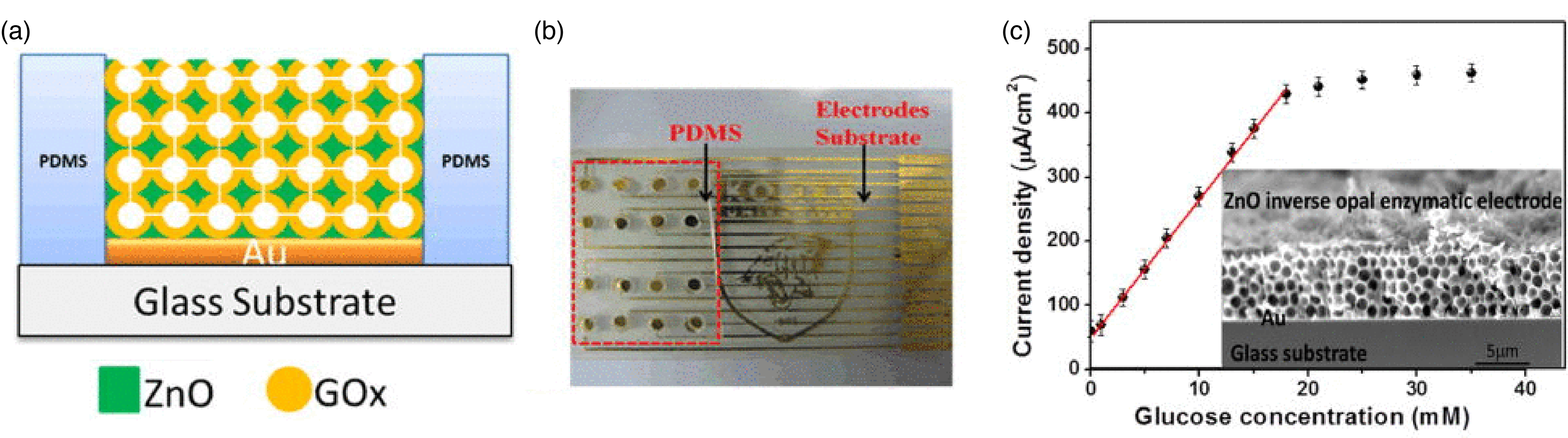
References:
[2] James H. Pikul, Paul V. Braun, and William P. King, “Micromechanical devices with controllable stiffness fabricated from regular 3D porous materials”, Journal of Micromechanics and Microengineering, vol. 24, 105006, 2014.
[1] Xueqiu You, James H. Pikul, William P. King, and James J. Pak, “Zinc oxide inverse opal enzymatic biosensor”, Applied Physics Letters, vol. 102, pp. 253103-5, 2013.